How the Right Technology Can Help Australian Researchers Meet the Present Urgency Surrounding a COVID Vaccine
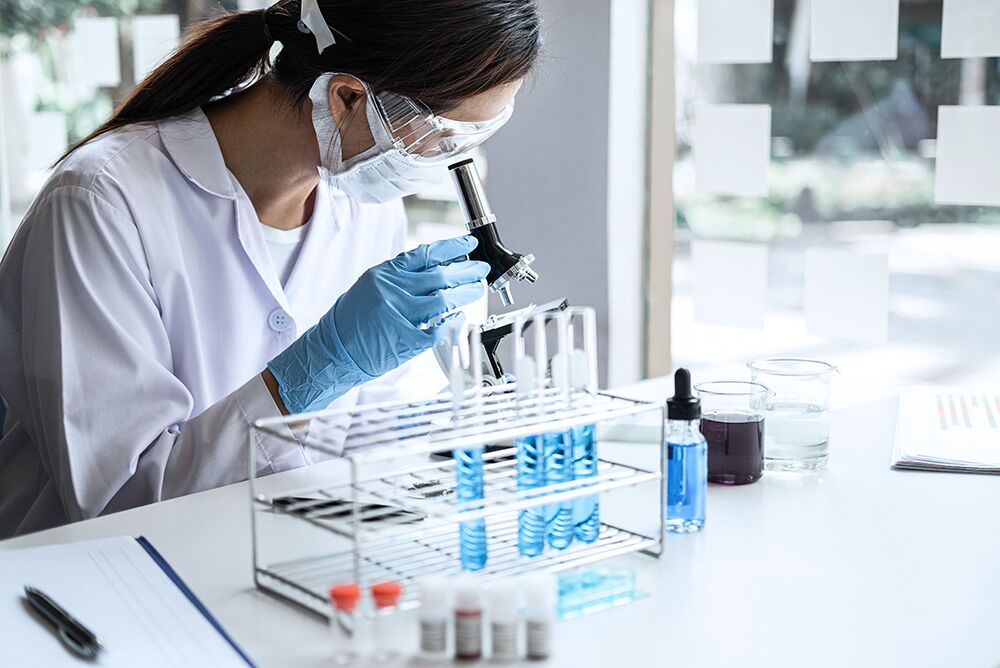
Vaccine development is a complicated process that can span a decade or even longer. Typically development and testing involve both the private and public sectors and procedures, while standardisation and regulations involve governments that also provide oversight. The process of vaccine development has similarities to early efforts, but today scientists understand viruses and diseases better than they did three hundred years ago. Advancements in stability, messenger RNA, live attenuated viruses, and delivery systems help fight mass infections such as those seen recently with COVID-19.
History of vaccine development and what scientific approaches have changed
As we interpret them today, vaccines are far from a novel concept. Vaccinae, a Latin word, is derived from vaccinus (of or relating to cows), based upon vacca, or cow. Vaccine arose to describe the cowpox material used for injections. The injection itself was called vaccination. The New England Journal of Medicine suggested this could be a misnomer as the vaccine was likely derived from Horsepox1. Widely acclaimed as the mastermind for his discovery in 1796, Edward Jenner’s findings came 22 years after Benjamin Jesty inoculated his children in 1774 in Dorset, UK. There is earlier evidence dating back to the 1500s. As early as 200 BCE, the scabs of the infected were ground down and blown into the nostrils of people in China.
The fundamental scientific approach has remained the same throughout history: identify, isolate, dose, and trigger an immune response. What has changed is the identification methods instead of relying on the symptoms. Genomic sequencing is ubiquitous in identifying the pathogen, while at the same time, drug formulation has broadened to encompass genetic modification and dosing beyond simple intramuscular injection.
What goes into a vaccine formulation?
Vaccine formulation is as varied as the pathogens it attempts to combat. The components have similar classifications such as antigens, stabilisers, adjuvants, antibiotics, and preservatives. The four pillars of a successful mRNA vaccine program are antigens, vectors, delivery (nanoparticle), and manufacturing2. Ultimately the desired outcome is the same: immunity.
Exploring the meanings of these terms helps in understanding their role. Consider an antigen as something that elicits an immune response; your body doesn’t recognise it and uses antibodies to rally the troops to kill off the invaders; it is the target. Stabilisers, such as sugars or oils, help prevent the vaccine from sticking to the vial or the syringe. Stabilisers also help the vaccine sustain its effectiveness during transportation and storage. Assisting the antibody to trigger the innate immune cells, such as macrophages, neutrophils, dendritic cells, or basophils, is an adjuvant. The adjuvants’ role is to heighten the response and elevate the reaction, thereby enhancing the antibody number. This enhancement is vital as the trickier bacterial and viral pathogens can slip through the first line of defence, and where our adaptive or acquired immunity uses T-Cells and B-Cells. These lymphocytes can take some time to develop as they have to learn about the invaders, but once known they remember the invaders in subsequent attacks. Added antibiotics prevent the vaccine from being infected by bacteria or fungi during manufacturing. Purification processes remove most of the antibiotic; however, trace quantities can remain. Not suitable for this purpose are antibiotics with track records of allergic reactions.
Addressing the stability and safety of vaccines
The ability to predict vaccine stability and efficacy early in the pipeline can help streamline development and optimise processes to identify the most efficacious and safe vaccine formulation. Early removal of potentially problematic molecules reduces time and costs, from vaccine formulation to manufacturing and quality control, and enables a more rapid response during pandemic times.
Formulation science has such a vital role in developing vaccines, yet many drug candidates fail the first couple of clinical trials due to an unstable formula. This instability may render the active ingredient inactive or alarmingly, create a toxic compound. The excitement of discovery can obfuscate the requirement to balance all the components, with innovators missing key formulation insights and low funding impeding research and access to cutting-edge expertise. Juggling temperature, pH, ionic strength, and cold chain distribution rely on highly skilled scientists understanding all the ingredients’ chemical interactions. It brings together pharmaceutical and materials science, protein engineering, and medicinal chemistry.
Creating a stable liquid formulation has challenges. Nature did not design proteins and peptides to last as long as a vaccine’s shelf-life dictates. The standard 2oC – 8oC temperature range is difficult to maintain in countries where the ambient temperature is beyond 40oC and access to cold chain infrastructure is out of the financial reach. Instability necessitates alternate modalities to improve shelf-life, storage, and transportation options.
Freeze-drying or lyophilisation is a process where a sample is frozen then subjected to a deep vacuum, well below the triple point of water. A small amount of heat energy causes any ice in the product to sublime, forming a powdered cake. This process is not a simple optimisation as often a lyophilised product requires excipients to add bulk and stability to the formulation. The process is prone to physical issues such as thermal collapse and insufficient freezing. Vaccines made initially from live attenuated microorganisms are being replaced by highly purified recombinant antigens that reduce reactivity but lack immunogenicity requiring the implementation of an adjuvant. Optimisation of adjuvants needs to continue to enhance its effectiveness in immune responses and remove undesirable reactogenicity.
What goes into vaccine characterisation?
Physicochemical analysis solutions offer scientists a way of understanding a vaccine formulation’s key characteristics and behaviour through the development pipeline and supporting manufacturing processes. When designing a vaccine, one must consider critical attributes such as thermal stability, sample homogeneity, viral titer, colloidal stability, particle size and concentration, adjuvant suitability, and polysaccharide composition to name a few.
In vaccine formulations, the ability to predict biological molecules’ stability is critical in ensuring their therapeutic efficacy and immunogenicity. Unstable biological molecules tend to undergo denaturation and aggregation. Protein aggregation in vaccines is of particular concern, reducing product efficacy and stability and increasing immunogenic risk. Influencing factors can be molecule concentration, pH, salt, temperature changes, or agitation level. Hence, it is essential to monitor the molecules throughout the development process carefully; from research, formulation development, and process monitoring to batch release.
By accurately characterising the various types of vaccines produced, we can better understand their structure and apply specific formulation modifications to control their behaviour and ability to trigger an immune response.
Messenger RNA (mRNA) based vaccines
Messenger RNA (mRNA) based vaccines encapsulated in a lipid nanoparticle have recently attracted considerable attention for their use in the COVID-19 pandemic. Recently, two mRNA vaccine candidates — one from Pfizer and BioNTech and another from Moderna — won emergency approval from regulators in several countries to fight COVID-19.
Traditional vaccines typically use weakened pathogens or fragments of the proteins or sugars on the surfaces known as antigens are injected into a patient to train the immune system to recognise an invader3. To create these vaccines, fertilised chicken eggs, cell cultures, or recombinant technology is used.
RNA vaccines are produced in the laboratory from a DNA template using readily available materials that are less expensive and faster than conventional vaccine production. RNA vaccines carry only the directions for producing these antigens. In this way, RNA is delivered into cells to allow the body’s cells to produce antigens and fight the infection. However, RNA is a fragile molecule that rapidly gets degraded by enzymes once inside our bodies. Encapsulating mRNA in a lipid nanoparticle helps overcome this challenge and ensures that a vaccine can successfully enter cells and deliver the mRNA into the cytoplasm.
Live attenuated virus
Live attenuated virus vaccines use a weakened form of the virus by passing it through animal or human cells until it picks up mutations that make it less able to cause disease. Vaccines for viruses such as influenza and newer applications such as dengue or respiratory syncytial virus (RSV) are part of this group, stimulating a strong, effective, and long-lasting immune response. Inactivated virus vaccines, such as influenza and polio vaccines, use chemicals or heat to render the virus uninfectious. However, large quantities of infectious viruses are required to make them. Inactivated vaccines tend to produce a weaker immune system response than live-virus vaccines. The addition of adjuvants and multiple doses can provide an effective immune response against the virus. VLPs or Virus-Like Particles are self-assembled viral protein complexes usually produced by bacteria or yeast and used to deliver genes or other drugs. Since VLPs can’t replicate, they offer a safer alternative to attenuated virus vaccines. Gardasil is one example of a VLP vaccine used to protect against human papillomavirus (HPV). Glycoconjugate vaccines, such as those used for meningococcal disease, combine a protein to a sugar glycan antigen to elicit a more robust immunological response and prevent bacterial infections.
Considerations for developing or formulating an RNA vaccine
While non-viral nucleic acid delivery systems enable revolutionary treatments such as mRNA vaccines, immuno-oncology, targeted oncology, CRISPR/Cas9 gene editing, and the treatment of rare diseases, a substantial need to encapsulate, protect, and deliver these payloads into diseased cells remains. Current production methods use crude pipette-mixing methods to perform nanoprecipitation of complex formulations that offer little control. They are operator-dependent and highly variable, all of which affect scalability and the potential to advance through manufacturing.
More recently, NxGen microfluidic mixing technology adopted by the NanoAssemblr platform offers time-invariant conditions that allow self-assembly to remain consistent throughout a single formulation and between individual formulations. This process enables volume-scaling of products across several orders of magnitude to suit various development stages. Because the Spark, Ignite, Blaze, and GMP systems share the same NxGen microfluidic architecture, rapid and easy process scaling from discovery to commercial production is possible.
An organic solvent containing dissolved lipids and an aqueous solution containing nucleic acids are injected into the two inlet channels of the NanoAssemblr cartridge. Under laminar flow, the two solutions do not immediately mix, but microscopic features engineered into the channel cause the two fluids to intermingle in a controlled and reproducible way, where molecules interact with each other by diffusion.
The two fluids are entirely mixed within milliseconds, causing a change in solvent polarity that triggers the homogenous self-assembly of nanoparticles loaded with nucleic acids. PNI offers a lipid library for vaccine applications suitable for intramuscular vaccination, making them more practical to administer than intravenous injections. PNI’s lipid nanoparticle (LNP) technology enables a synthetic vaccine without the complications of a packaging cell line, contamination with a replication-competent virus and anti-vector immunity. LNP formulations are used for all RNA vaccines currently being developed for COVID-19 because they offer a desirable alternative to viral delivery.4
Characterisation of the encapsulated drug for improving the fundamental understanding of nucleic acid delivery systems
Malvern Panalytical (MP) extends the analytical toolbox to characterise vaccine products, overcoming the challenges associated with traditional methods. MP has developed new analytical techniques for vaccine formulation and implemented a systematic analytical quality by design approach to ensure the right process for the right purpose.
Below are a few of the physicochemical characterisation tools that deliver information essential to ensuring the vaccine product’s stability and efficacy, from the initial characterisation of biological materials to final manufacturing and quality control.
Differential Scanning Calorimetry (DSC)
DSC enables understanding and monitoring of proteins’ structure and thermal stability at every stage of virus-based vaccine development and during process development for recombinant products. DSC is also used to understand the thermal stability of liposomes used as carriers in nucleic acid-based vaccines. See MicroCal PEAQ-DSC.
Isothermal Titration Calorimetry (ITC)
ITC is a technique used in quantitative studies of biomolecular interactions. It works by directly measuring the heat that is either released or absorbed during a biomolecular binding event. ITC goes beyond binding affinities and can elucidate the mechanisms underlying molecular interactions. This more in-depth understanding of structure-function relationships enables more confident decision making during vaccine development. See MicroCal PEAQ-ITC.
Electrophoretic light scattering
Electrophoretic light scattering helps characterise and formulate product development such as mRNA vaccines that use virus-like particles (VLPs), liposomes, and other nanoparticles as carriers to determine the size and colloidal stability. Zeta potential is a measure of intermolecular electrostatic interactions. Higher zeta potential increases repulsion amongst molecules, minimising aggregate formation. Protein aggregation is of particular concern in formulations as it can reduce vaccine stability and efficacy and increase immunogenic risk. See Zetasizer Ultra.
Dynamic light scattering and laser diffraction
These tools enable the measurement of particle size and size distribution to detect aggregates’ presence and ensure sample homogeneity in the development of vaccines of all types. See Zetasizer Ultra and Mastersizer 3000.
Depending on the particle size involved, Multi-Angle Dynamic Light Scattering and Nanoparticle Tracking Analysis are used to measure viral titer throughout the development lifecycle for virus-based vaccines. See NanoSight.
Particle concentration is critical when using carriers such as VLPs, liposomes, and other nanoparticles. Nanoparticle Tracking Analysis and Multi-Angle Dynamic Light Scattering measure particle concentration during characterisation and formulation development stages. See NanoSight and Zetasizer Ultra.
Many vaccines require an adjuvant in the formulation to ensure an effective immune response. Depending on the vaccine type, Electrophoretic Light Scattering, laser diffraction, Nanoparticle Tracking Analysis, and Differential Scanning Calorimetry all have uses in optimising formulation with adjuvants. See Zetasizer Ultra and MicroCal PEAQ-DSC.
Size exclusion chromatography
Size exclusion chromatography offers advanced detection aids in the compositional analysis of the protein and polysaccharide content of polysaccharide-conjugate vaccines during process development and manufacturing. See Malvern OMNISEC GPC/SEC.
Circular Dichroism (CD) spectroscopy
This tool helps study protein conformation and stability and the effects of manufacturing, formulation, and storage conditions on vaccine performance. Scientists can use it to determine chiral drugs and proteins’ stereochemistry and monitor and characterise molecular interactions in solution. Although both DSC and CD spectroscopy can measure thermal denaturation of proteins, CD uses lower concentrations of proteins than DSC and can also be measured at various pHs and in a broader range of solvent conditions. See Jasco J-1500 CD.
Microfluidic diffusional sizing (MDS)
A novel technology that characterises proteins and interactions in solution works under native conditions and based on physical properties that determine function. MDS eliminates complications associated with surface-based measurements such as binding artefacts or non-specific protein adsorption. It also enables researchers to obtain valuable information about binding targets they cannot get using other technologies. This approach allows rapid evaluation of affinity and concentration for a complete immune response assessment, directly in complex solutions such as serum. See Fluidity One-W.
For more information about developing and formulating vaccines, speak with ATA Scientific today.
References:
1. A. Nitsche et al., The New England Journal of Medicine (11 October 2017) ©2017 Massachusetts Medical Society
2. NanoMedU EPC 102V Infectious Diseases Shell Ip, PhD Precision Nanosystems https://www.precisionnanosystems.com/
3. Nature 589, 189-191 (2021) https://www.nature.com/articles/d41586-021-00019-w4. RNA Platform, Precision Nanosystems https://www.precisionnanosystems.com/platform-technologies/rna-platform